(Jump to Our Projects)
What we study and Why
The University of Rochester Neutrino Group works on a variety of experiments designed to study the masses of fundamental particles called neutrinos. Neutrinos have very small masses compared to other known fundamental particles, and display a strange phenomenon known as neutrino flavor oscillation. This occurs because the “flavors” of neutrinos, the neutrinos that interact with the electron and heavier siblings, the muon and the tau, are mixtures of at least three neutrinos of definite masses.
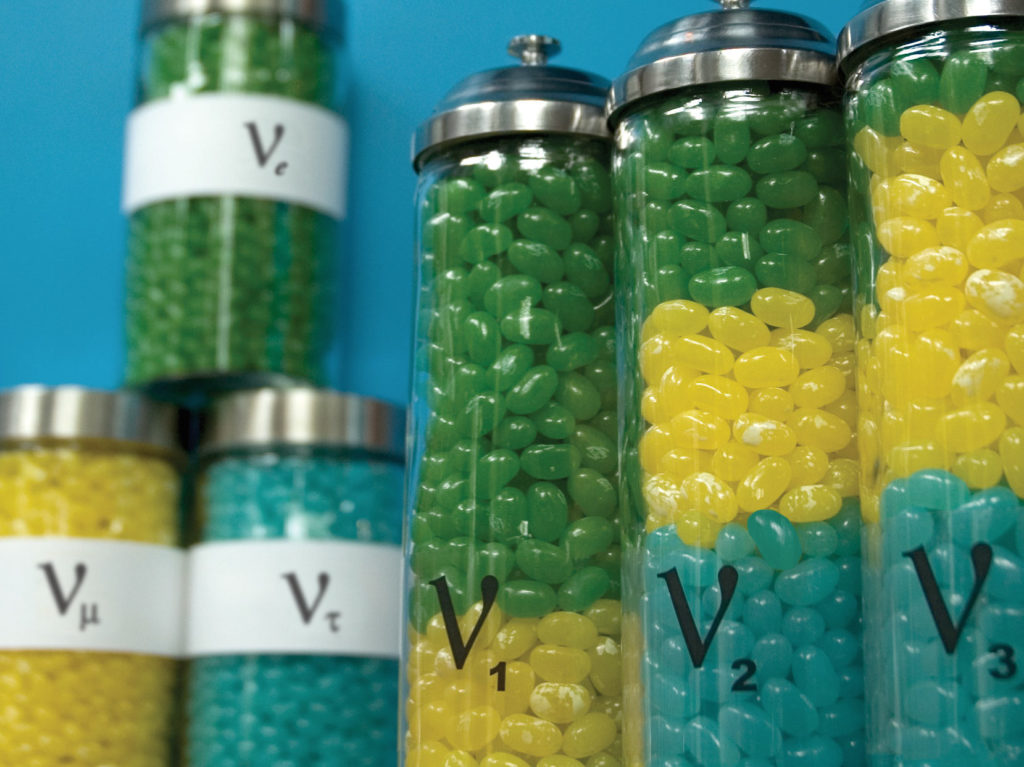
One idea is that small differences in oscillation between neutrinos and their anti-matter partners, “anti-neutrinos”, may be a manifestation of the same mechanism that led to today’s universe being dominated by matter rather than the equal parts of matter and anti-matter produced in the big bang. Our experiments seek to find those small differences in oscillations.
(Photo: An illustration of the three neutrino mass states and the three flavors that make them up (electron, muon and tau). Image: Fermilab/Sandbox Studios.)
DUNE
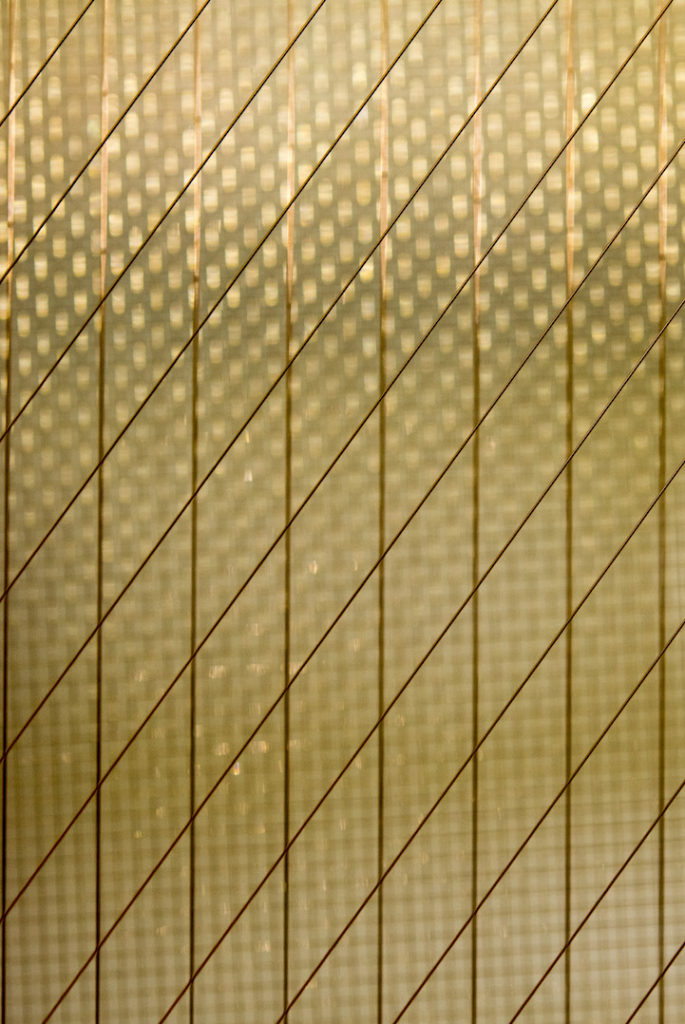
DUNE, the Deep Underground Neutrino Experiment, is an experiment currently in construction and planning to run at the long baseline neutrino facility at Fermilab. It includes also a 40,000 ton liquid argon detector in the former Homestake Gold mine in Lead, South Dakota, and a near detector for neutrino monitoring at the Fermilab site. It is a multi-national experiment that plans to write the book on neutrino masses and mixing, including the possibility that neutrinos and antineutrino have different oscillations in vacuum. The experiment is already a decade into planning, and is the culmination of a long line of research, including our other neutrino projects. First data is expected in 2028. The Rochester group works primarily on the near detector.
SBN
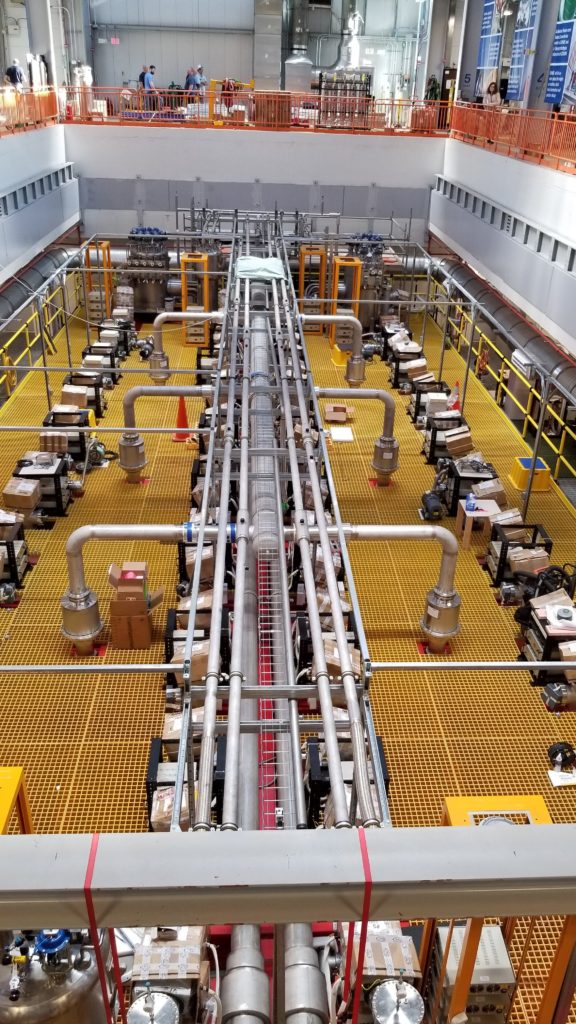
The SBN experiments at Fermilab are studying the possibility that neutrino oscillations occur with low probability over proper times much shorter than the known neutrino oscillations in nature. The detectors used in the experiment are liquid argon TPCs, similar to the technology of the DUNE experiment. The near detector, SBND, is currently under construction. We work on the far detector, ICARUS, which is being commissioned and taking first neutrino data in 2020 and 2021. Our interests include using our knowledge of neutrino interaction physics to study and build improved energy estimators and background rejection algorithms for electron neutrino reconstruction in ICARUS.
T2K
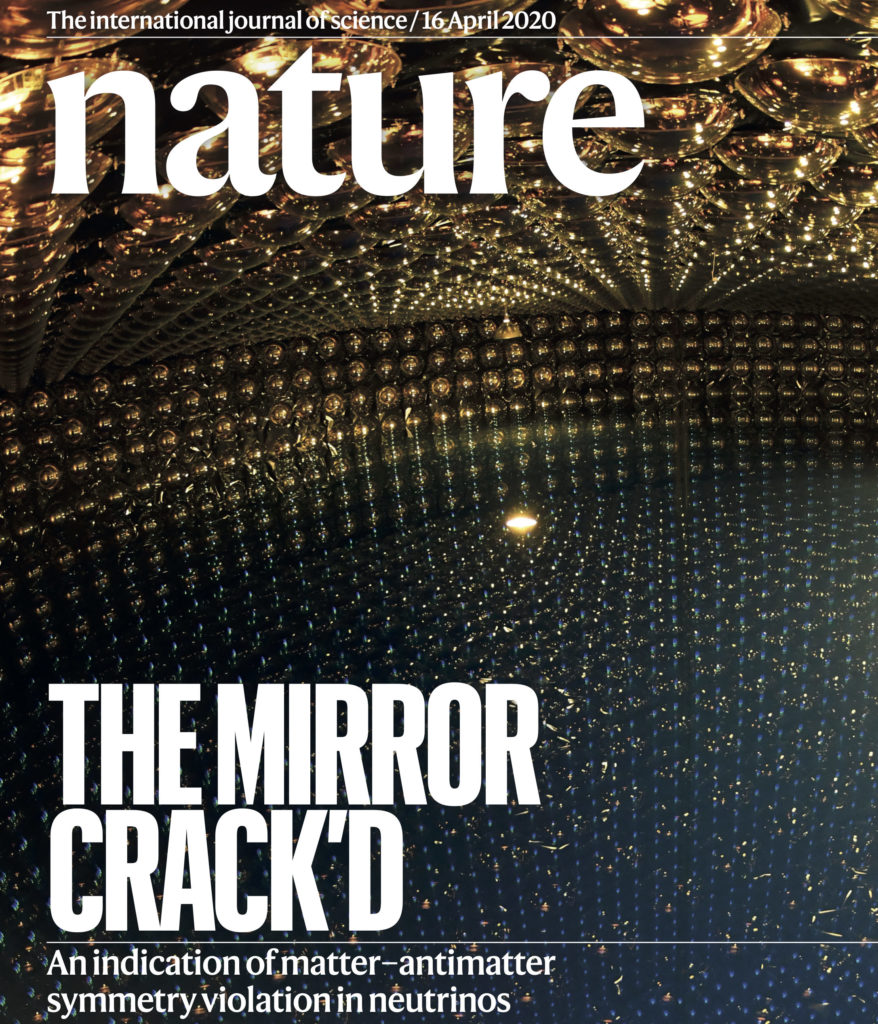
T2K (Tokai to Kamioka) is a neutrino oscillation experiment in Japan. We produce a neutrino beam at J-PARC on the east coast of Japan, and observe it near the west coast of Japan in cavern in a former zinc mine which houses the Super-Kamiokande detector. The long distance over which the neutrinos travel gives them time to oscillate. T2K has produced a hint of the neutrino-antineutrino oscillation differences that we wish to study, and continues to run and analyze data. We work primarily on adding new samples to the neutrino oscillation analysis and applying what we learn about neutrino interactions to improve the studies.
MINERvA
The MINERvA experiment ran at Fermilab from 2008-2018. It seeks to measure in detail how neutrinos interact on the atomic nuclei in neutrino detectors. Understanding those interactions is important for the precision experiments required to understand small differences in neutrino and antineutrino oscillations. MINERvA was the first experiment to demonstrate the importance of correlated pairs of neutrons or protons inside the nucleus in nearly elastic scattering from nuclei. We continue to analyze data from MINERvA and are working to “preserve” those measurements in a way that will be useful for future studies.
Neutrino Interaction Phenomenology
Neutrino oscillation and interaction experiments use models to describe the particles created when a neutrino interacts in our detector. Because neutrino oscillation experiments measure energy from the observed final state particles, this model is a key tool for understanding the performance of the experiment and uncertainties in the analysis. The models that we use, particularly those that describe the influence of the nucleus on neutrino scattering processes, are necessarily approximate because of the complexities inherent in the strongly coupled nuclear system. Our group works on a number of problems related to this modeling. Recent work includes: extraction of nuclear potential effects from electron scattering for quasielastic interactions and pion production, extraction of form factors from electron and neutrino scattering data for nucleons and Δ resonances, parametrization of nuclear potentials and deep inelastic scattering with simple, effective models, and asymmetries in pion-nucleon correlations in neutrino scattering.